Which metals are critical for green energy?
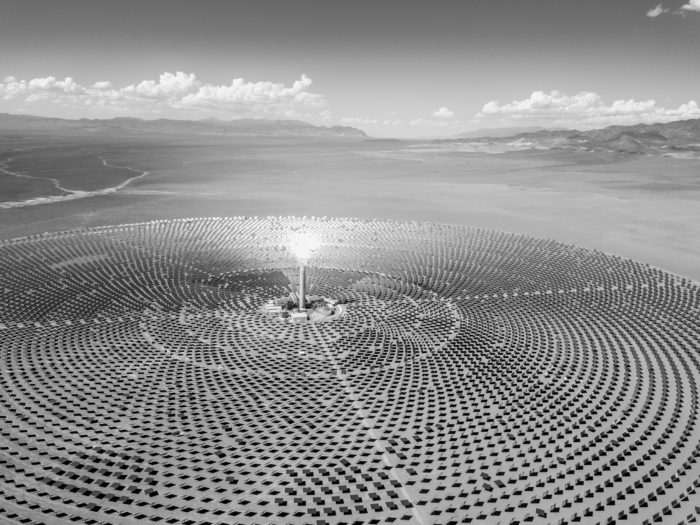
Low-carbon technologies require more raw materials than traditional technologies. For example, manufacturing a traditional combustion engine vehicle requires 20 kg of copper, compared with 40 kg for a hybrid vehicle and with 80 kg for an electric vehicle.
Renewables demand a great deal of metals in general. Base metals such as aluminium, copper or manganese are widely used in green tech, but this consumption represents only a small part of their total final consumption. In contrast, some rare metals are essential for clean energy technologies and their application to those industries can represent a significant proportion of their total final consumption.
The multiplicity of different technologies used within a single green sector also entails the need for a plethora of metals within a single renewables industry.
The three main technologies for solar energy, for example, reflect this fractalised rare metals demand :
- Silicon panels – the most widespread product. These panels can be monocrystalline or polycrystalline.
- CdTe thin films (Cadmium Telluride).
- CIGS thin films (Copper – Indium – Gallium – Selenium).
The metals used in wind turbines also depend on which specific technology is in play. Some wind turbine rotors contain permanent magnets, composed specifically of neodymium as well as terbium, dysprosium and boron. These permanent magnets are the least common in wind farms currently, but they are increasingly used in new wind turbines because they combine several advantages: low maintenance, weight reduction, longer life as well as higher efficiency. These advantages are particularly attractive for offshore wind.
The various turbines used in several renewable energy sources (hydraulic, wind, and geothermal) also require metal alloys containing small quantities of rare metals – molybdenum for example.
Finally, the transport and storage of the energy produced in these industries requires large quantities of copper, which could be the base metal most subject to criticality in the years to come. But very large quantities of lithium, cobalt and nickel are also required for batteries. Demand for cobalt could triple by 2030 whilst this metal is subject to geopolitical tensions linked to the political instability of the Democratic Republic of Congo, the world leader in cobalt production. Demand for lithium could quadruple in that same timeframe.
Investing in critical green energy metals
The amount of money invested in renewable energy was USD 330 billion in 2018 according to Bloomberg New Energy Finance, shared mainly between solar (USD 130 billion), and wind (USD 128 billion), thanks to a surge in offshore wind farms. The biomass and biofuels industries, although more modest with USD 6 and 3 billion respectively, are also in strong growth: + 18% and + 47% respectively compared with 2017.
According to the International Energy Agency (IEA), nearly 170 GW are expected to be added in 2020 to the production capacity of renewable energies, down from 2019 because of COVID-19. However, the IEA expects another record year in 2021 with an addition of capacity approaching 200 GW, driven by China (40%), Europe (16%) and the United States (12%). While the boom in renewable energies is undeniable, the uncertainty lies in the technological choices that will be made for their development, involving greater or lesser quantities of specific metals.
The variety of solar panel types is a case in point, because as in any energy sector, greater efficiency is always sought at a lower cost. First generation silicon panels today still represent 90% of the market, because despite a relatively low efficiency, they are cheap and the silicon that composes them is abundant (though these panels do contain silver or nickel as well as copper, to a lesser extent).
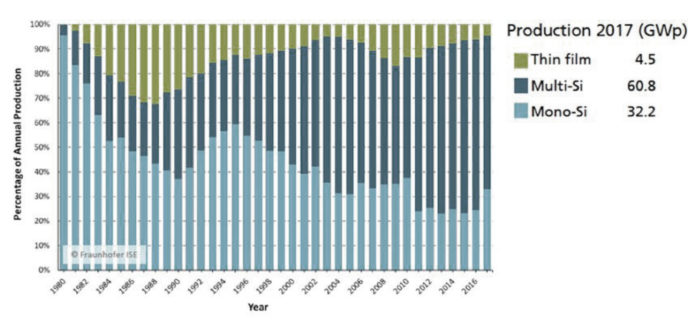
Source : Fraunhofer Institute for Solar Energy Systems
Thin-film solar panels require lower amounts of raw material, but they are made of metals that are rarer and more expensive than silicon. Thus, CdTe panels combining cadmium and tellurium presented themselves as an interesting alternative, and are currently the second most widespread panels. However, these panels are copper-heavy, are toxic (Cadmium), and rely on metals that are rarer than silicon. CdTe-type thin-film photovoltaics therefore did not experience the significant development that some had predicted.
Nevertheless, CIGS-type thin-film solar cells containing copper, indium, gallium and selenium may become more common in the years to come because they are less toxic, more economical in terms of raw materials and have better efficiency. Developments in the CIGS cell market therefore deserve to be followed. CIGS cells require 13 tons of indium, 4 tons of gallium as well as 41 tons of selenium per GW, which is significant compared with the annual production of these metals, as indicated below:

Some third-generation technologies are in development and could use more abundant organic elements (carbon, oxygen, hydrogen) or other metals such as platinum, ruthenium and cobalt. However, it will be difficult to supplant existing technologies, and in particular silicon panels, as they are now deployed on a very large scale and at low cost.
The development of concentrated solar thermal power plants, another alternative source of solar energy, would require significant amounts of copper, nickel and silver. This type of power station concentrates the sun’s rays using mirrors to heat a heat transfer fluid, and then heat energy is transformed to produce electricity.
Solar technologies are therefore more and more competitive, and each technology is the subject of intense development activity to increase the efficiency of cells and improve their durability as well as to refine them so as to use less raw material for any given energy output. Currently, around 100 GW are added each year to the global photovoltaic park.
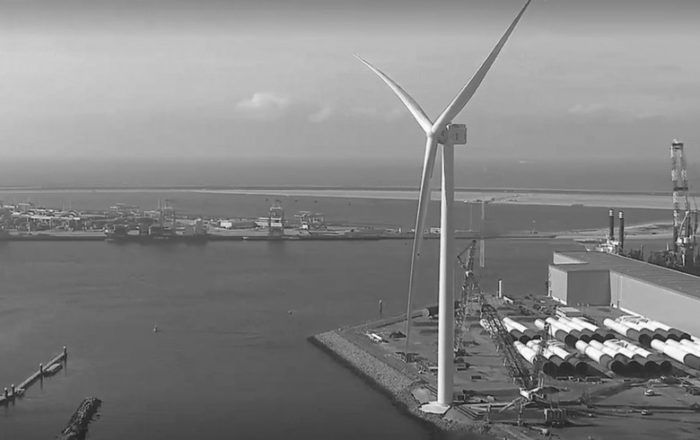
Wind power is also showing significant development, with a 43 GW increase in global capacity in 2018. Offshore wind power is also receiving renewed interest with the development of ever more imposing and powerful wind turbines. In 2018, offshore turbines had an average capacity of 5.5 MW each, with an average rotor diameter of 150 meters. The latest turbine from General Electric, named Haliade-X, has a capacity of 12 MW for a span of 200 meters!
Onshore wind power is no exception to this growth trend either, and could triple its current capacity by 2030. This growth is explained by increasingly low installation costs as well as the impetus of the Asian market, particularly in China and in India. Another critical issue for wind power is the ability to maintain wind turbines and replace turbines that are too old or defective. Indeed, by the end of 2020, 28% of European turbines will have exceeded fifteen years of service.
Thus, the development of wind power should drive the demand for permanent magnets, particularly for off-shore wind turbines for which weight and maintenance are more fundamental constraints, as is the demand for specialised steels used for the mast and the nacelle (steel represents about 80% of the mass of a wind turbine).
Specialised steels will also be required for various hydraulic and geothermal technologies.
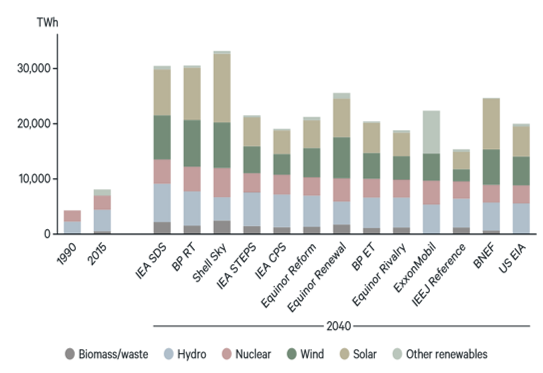
Source : Resources for the Future, Global energy outlook 2020
The graph above highlights the trend – shared by the different scenarios – for the spectacular increase in electricity production from solar and wind energy, and more generally the progression of different renewable energy industries by 2040.
However, the promise of a significant boom in renewable energy tech should not obscure the need to anticipate the intermittency of energy production from renewable sources, in order to ensure reliable access to renewable energy. We will need to exploit the complementarity of solar and wind power (diurnal and seasonal respectively), accommodate the irregular output from methanation and from PSPSs (Pumped Storage Power Stations), and manage different storage technologies (batteries, hydrogen, compressed air): So many major challenges involved in making the development of sustainable energies a sustainable process in itself.
Therefore, smart electricity grids, or “smart grids,” have the purpose of optimising and smoothing the production of energy sources that have become integral to a nation’s power consumption. Smart grids collect information about the state of a grid in order to adjust the flow of electricity in real time and enable efficient grid management. Smart grids require significant computing power but make it possible better to exploit the potential of green energy tech, on a local but also on a continental scale, by cooperating at the level of the European network, for example.
And so the production of renewable energies is not the only area of our energy transition that will require critical metals. Our various green energy storage technologies, in particular, will be full of rare metals: Batteries, depending on the technology, will use lithium, nickel and lead, and we’ll also need more zinc and vanadium for redox batteries. The transformation of renewables also requires rare metals: The electrolysis of water to produce the promising non-polluting fuel hydrogen is catalysed with platinum, and in the future may be catalysed with the less expensive platinum group metals, including ruthenium.
The proliferation of computer equipment accompanying the development of these energies also requires many rare metals such as silver, ruthenium and tantalum, to name a few. The demand for base metals such as copper, iron and aluminum will also increase significantly.
However, the development of renewable energy sources is, first of all, dependent on favourable energy policies. Indeed, giving ourselves the means to meet the 2°C global warming bar stipulated in the 2015 Paris Agreement involves a major energy shift. Consequently, the level of demand for rare metals could be as generally forecasted, or double those forecasted amounts, depending on the various possible scenarios for the energy transition.
Green energy is still a long way from supplanting fossil fuels. Nevertheless, it is reasonable to predict a marked growth in sustainable energy in the years to come, accompanied by a significant increase in the demand for the rare metals that are critical for those energy sources. These rare metals therefore all present investment opportunities that deserve to be closely monitored.
Sources
- Carbon Brief, Explainer: These six metals are key to a low-carbon future, 2018.
- Center for climate and energy solutions, https://www.c2es.org/content/renewable-energy, 2019.
- Institute for Sustainable Futures, Responsible minerals sourcing for renewable energy, 2019.
- International Renewable Energy Agency (INERA), Future of Wind, Octobre 2019.
- André Månberger, Björn Stenqvist, Global metal flows in the renewable energy transition: Exploring the effects of substitutes, technological mix and development, 2018.
- Wind Europe, Financing and investment trends report, 2019.
- Richard G. Newell, Daniel Raimi, Seth Villanueva, and Brian Prest, Resources for the Future, Global energy outlook 2020: energy transition or energy addition?, mai 2020.
- Encyclopédie de l’énergie, https://www.encyclopedie-energie.org, 2019.